It is the Rectus Femoris (RF) that is the most prevalent muscle injured within the quadriceps complex (1, 2) and although hamstring tears have been shown to occur more frequently within a season, RF tears have a significantly higher (p<0.01) incidence rate (29%) compared to hamstring tears (11%) during preseason (2). With several intrinsic and extrinsic risk factors involved in muscle tears it is previous injury that is consistently the highest risk factor for injury (3). The objective of this post is to provide the strength and conditioning coach with the underlying biomechanical mechanisms of RF injury in football with a view to aid exercise selection for injury prevention strategies.
RF anatomy has been suggested to contribute to its injury risk (4). Its characteristics of a fusiform, predominately type II muscle fibered (65%) bi-articular muscle designed to produce significant length changes and high shortening velocities in knee flexion and hip extension, make it susceptible to high eccentric demands thus injury (4, 5, 6). It is kicking, specifically the instep kick on the dominant or preferred kicking leg that is the most common mechanism for RF injury in football (4, 7). Controversy exists within the literature as to whether RF tears occur during the swing phase or ball contact of kicking (4). Therefore, a kinematic and kinetic analysis of the instep kick must be performed.
Instep kicking kinematics can be divided into three phases dictated by four key moments as can be seen in figure 1 (8). The kicking leg is further broken down into two segments, the proximal segment the thigh and the distal segment the shank and foot (9).
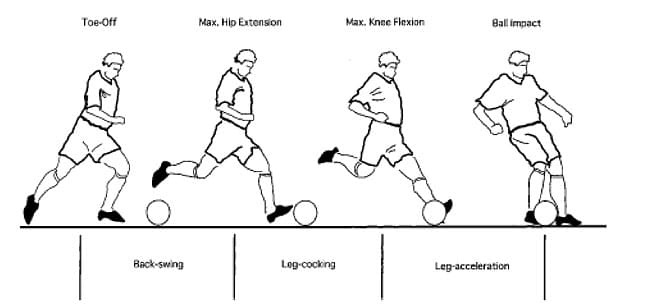
PHASE 1
The Back Swing: Defined as the period from right toe off to the time maximum hip extension is achieved, whilst knee flexion is occurring (8).
During late back swing:
- Hip extension reaches 29° with adduction and external rotation at an angular velocity of 171.9 – 286.5 deg·s -1 (8,10).
- The knee flexes and internally rotates at an angular velocity of 745 – 860 deg·s -1 and the ankle is plantarflexed (10°), abducted (20°) and slightly pronated, reaching a maximum angular velocity of 860 deg·-1 (8,10).
- Electromyography (EMG) results show at this stage of the kicking motion the RF has a mean activation of 47.8% (11).
- Total time spent in the back swing is the longest phase at 108ms, contributing to 48.5% of the total duration of the instep kick (8).
PHASE 2
Leg Cocking: Subsequently follows on from the back swing and ends with maximal knee flexion (8).
During leg cocking
- The proximal segment initiates movement and sees the thigh angular velocity become positive at 286-401 deg·s -1 (8).
- However the shank angular velocity continues to be negative until maximal knee flexion is achieved of 113° at an angular velocity of 745 – 860 deg·s -1 (8, 12, 13, 14).
- EMG at this stage shows an 85.5% RF mean activation level (11).
- Hip flexion (249 N.m) and knee flexion (98 N.m) torques are reached during the leg cocking phase just prior to the initiated motion of knee extension (8).
- The leg-cocking phase lasted 62ms, which contributes to 28% of the total duration of the instep kick (8).
- The transition between the leg cocking phase and the acceleration phase utilizes the stretch shortening cycle that sees a stretch of the knee extensors during back swing followed by a rapid shortening to accelerate a positive shank movement (15).
PHASE 3
Leg Acceleration: Is where knee extension occurs and continuously extends until ball impact (8).
During leg acceleration
- As leg acceleration continues the thigh reaches its maximum peak value (516-573 deg·s -1) before knee extension begins, as the knee extends angular velocity of the thigh decreases (9).
- Shank velocity increases linearly until ball impact where thigh angular velocity is zero and the shank and foot reach peak angular velocity (1891 deg·s -1) but zero acceleration (9, 12).
- Kinetic input to the leg system is not only generated from a single source (muscle force) but is a combination of both muscle moments and motion-dependent moments highlighting segmental interactions displayed in a proximal to distal sequence (9, 16).
- However, in the final stages immediately before ball contact, knee extension muscle moment rapidly decreases and begins to exhibit a reverse (flexion) moment despite the motion dependent moment continuing to increase displaying an extension moment at ball contact (17).
- It is speculated that as the angular velocity of the shank and foot exceed the inherent force-velocity limitation of the associated muscular system, it becomes incapable of generating any concentric force and therefore the reverse knee muscle moment is a resistance to a stretch of the muscular system (17).
- Evidence of a reverse knee muscle moment is supported as the biceps femoris peaks in activation levels at 40.1% just before ball impact via EMG (12).
- RF mean activation levels decrease to 59.1% (11).
- Temporal changes during leg acceleration show the phase lasted 51ms and constitutes 23.2% of the total kicking action (8).
Figure 2 demonstrates this detailed time-series change in angular velocity (17).
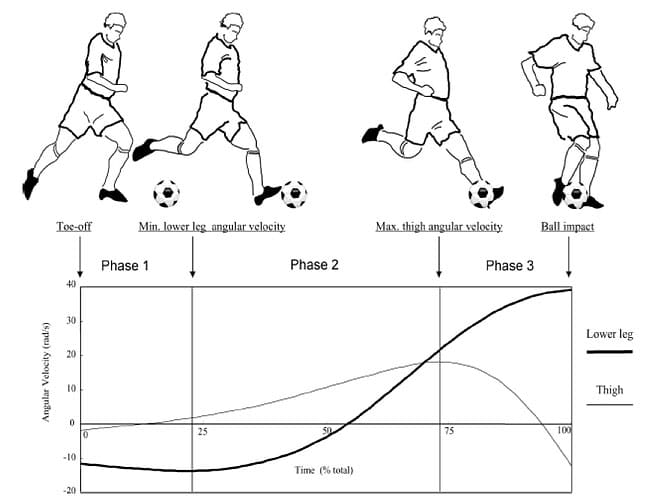
Muscle tears are stretched induced caused when active muscles are forced to lengthen too greater than optimal lengths during powerful contractions (18). Thus, the leg-cocking phase utilizes the bi-articular role of the RF that sees a significant eccentric contraction via a maximal length change in knee flexion. This is performed whilst the muscle is simultaneously producing peak hip flexion torque and therefore the highest levels of muscle activity throughout the whole kicking cycle are exhibited. This is compounded by the fast stretch shortening cycle moment that also produces peak knee extension torque representing a powerful contraction. In comparison, the ball contact phase sees a concentric only contraction from the RF that is actually inhibited just prior to ball contact that sees a knee flexion moment produced by the biceps femoris. Further, the activation levels of the RF are reduced and knee extension is dominated by the vastus lateralis during the final stages of the kicking cycle.
_________________________________________________________________________________________
From the above phase descriptions it appears clear that the RF is at the highest risk of tear during the leg-cocking phase of the back swing, during the swing phase of the instep kick. Thus, a potential mechanism for RF injury in football has been identified via a biomechanical analysis, which provides the strength and conditioning coach a platform to devise a mechanism specific, injury prevention strategy.
Strength training is an important component in the quest for injury prevention within football although the exact content that constitutes this component is still a topic of uncertainty and debate. To deliver a injury prevention program with a sound theoretical rationale, an understanding of biomechanics is required so the strength and conditioning coach can identify a mechanism of injury, understand the mechanical properties that need to be altered to prevent that injury and select an exercise that has specificity to the mechanism.
References
- Speer, K.P., Lohnes, J., & Garrett, W.E. (1993). Radiographic imaging of muscle strain injury. The American Journal of Sports Medicine, 21, 89-96.
- Woods, C., Hawkins, R., Hulse, M., & Hodson, A. (2002). The football association medical research programme: an audit of injuries in professional football – analysis of preseason injuries. British Journal of Sports Medicine, 36, 436-441.
- Arnason, A., Sigurdsson, S.B., Gudmundsson, A., Holme, I., Engebretsen, L., & Bahr, R. (2004). Risk factors for injuries in football. The American Journal of Sports Medicine, 32, 5S-16S.
- Mendiguchia, J., Alentorn-Geli, E., Idoate, F., & Myer, G.D. (2013). Rectus Femoris muscle injuries in football: a clinically relevant review of mechanisms of injury, risk factors and preventive strategies. British Journal of Sports Medicine, 47, 359-366.
- Hasselman, C.T., Best, T.M., Hughes, C., Martinez, S., & Garrett, W.E. (1995). An explanation for various rectus femoris strain injuries using previously undescribed muscle architecture. The American Journal of Sports Medicine, 23, 493-499.
- Johnson, M.A., Polgar, J., Weightman, D., & Appleton, D. (1973). Data on the distribution of fibre types in thirty-six human muscles: an autopsy study. Journal of the Neurological Sciences, 18, 111-129.
- Ekstrand, J., Hagglund, M., & Walden, M. (2011). Injury incidence and injury patterns in professional football: the UEFA injury study. British Journal of Sports Medicine, 45, 553-558.
- Nunome, H., Asai, T., Ikegami, Y., & Sakurai, S. (2002). Three-dimensional kinetic analysis of side-foot and instep soccer kicks. Medicine & Science in Sport & Exercise, 34, 2028-2036.
- Kellis, E., & Katis, A. (2007). Biomechanical characteristics and determinants of instep soccer kick. Journal of Sports Science & Medicine, 6, 154-165.
- Levanon, J., & Dapena, J. (1998). Comparison of the kinematics of the full-instep and pass kicks in soccer. Medicine & Science in Sports & Exercise, 30, 917-927.
- Manolopoulos, E., Papadopoulos, C., & Kellis, E. (2006). Effects of combined strength and kick coordination training on soccer kick biomechanics in amateur players. Journal of Medicine & Science in Sports, 16, 102-110.
- Dorge, H.C., Andersen, B., Sorensen, T., Simonsen, E.B., Aagaard, H., Dyhre-Poulsen, P., & Klausen, K. (1999). EMG activity of the iliopsoas muscle and leg kinetics during the soccer place kick. Scandinavian Journal of Medicine & Science in Sports, 9, 195-200.
- Lees, A., & Nolan. (1998). The biomechanics of soccer: a review. Journal of Sports Science, 16, 211-234.
- Levanon, J., & Dapena, J. (1998). Comparison of the kinematics of the full-instep and pass kicks in soccer. Medicine & Science in Sports & Exercise, 30, 917-927.
- Bober, T., Putnam, G., & Woodworth, G. (1987). Factors influencing the angular velocity of a human limb segment. Journal of Biomechanics, 20, 511-521.
- Lees, A., Asai, T., Andersen, T.B., Nunome, H., & T. Sterzing. (2010). The biomechanics of kicking in soccer: a review. Journal of Sports Science, 28, 805-817.
- Nunome, H., Ikegami, Y., Kozakai, R., Apriantono, T., & Sano, S. (2006). Segmental dynamics of soccer instep kicking with the preferred and non-preferred leg. Journal of Sports Sciences, 24, 529-541.
- Mueller-Wohlfart, H., Haensel, L., Mithoefer, K., Ekstrand, J., English, B., McNally, S., Orchard, J., Niek van Dijk, C., Kerkhoffs, G.M., Schamasch, P., Blottner, D., Swaerd, L., Goedhart, E., & Ueblacker, P. (2013). Terminology and classification of muscle injuries in sport: a consensus statement. British Journal of Sports Medicine, 47, 342-350.